Planetary Nebulae
Peter Sorensen
Planetary Nebulae are among the most beautiful objects in the sky and
show an amazing variety of shapes, sizes and brightness. They represent
the final stage of the vast majority of stars in the universe, and hence have
become one of the main research areas of modern astrophysics. At the
Roque de los Muchachos there are several astronomers working in this
field, making use of the telescopes to investigate many aspects of these
interesting objects. This article will give a brief overview of what we
know about Planetary Nebulae today, and also describe some of the
research we are doing at the observatory.
Planetary Nebulae (PNe) are not planets at all, and have nothing to do
with the formation of planets either. The name can be traced back to the
eighteenth century, when many of the objects in the sky were being
classified. The British astronomer, William Herschel, (after whom we have
named the 4.2m telescope) was a pioneer in this field. He carried out an
immensely large study, and named all objects which had the same characteristics as the
faint disks around the known planets for Planetary Nebulae. It was
believed that these objects either were planets or stars in the process of
being formed from a huge sphere of gas. The advent of spectroscopic
techniques marked the age of modern astrophysics. In the twentieth
century astronomers learned how to study the radiation from celestial
objects by splitting up the light into the different colours (or wavelengths),
much like we see the rainbow. All the different elements we know today,
like hydrogen, helium etc., emit or absorb light only on very specific
wavelengths. These 'fingerprints' can tell us a great deal about the
chemical composition of the light emitting source as well as give some
important information about the kinematics. For example, if a star is
moving towards us or moving away. Only with the development of these
techniques did astronomers finally reveal the true nature of the PNe, not
as planets but as the dying process of the majority of all stars.
A few decades ago, theoretical studies clarified that stars evolve and die
in different ways depending on the mass of the stars. A star is a sphere of
gas where the gravitational force, which tends to pull all the particles
together, is balanced by an outgoing pressure from the gas itself. This
pressure originates from the core of the star, where energy is constantly
produced in thermonuclear reactions, converting hydrogen into helium.
Our sun is presently undergoing such an evolutionary stage, where it will
remain for several thousand millions years to come. At the end of this
stage, also called the main sequence phase, things begin to become more
complicated. Stars as massive as the Sun (or a few times more massive)
go through a series of evolutionary stages, gradually burning more and
more heavy elements and in the end reaching what is called the
Asymptotic Giant Branch. At this stage, the star has expanded its
atmosphere many thousands times to a thin, red-glowing ball of fire. This
scenario is what we expect will happen to the Sun too, and will mean the
ultimate end of all life on Earth, as the planet will be enveloped in the
glowing fireball. Everything will burn up, and the seas begin to boil. The
core of the star, no longer able to produce energy, will collapse and heat
up until a point where all atoms basically touch each other. At that
moment, the density of the core will be so high that one spoon full of core
material easily could weigh as much as an elephant!.
This phase of the
star's evolution is however highly unstable, and the outer layers of the atmosphere begin to pulsate and loose mass to the interstellar media.
The instability becomes stronger and stronger, and finally causes the
ejection of a significant part of the star's mass in an expanding shell.
The stellar core remains as an extremely hot, small central star. At this
point, we see the beginning of a Planetary Nebulae. Initially, the core is
surrounded by dust which
prevents the light from escaping outwards. Later, this dust layer
disappears, and from the exposed core emerges ultra high energetic rays as
well as a fast wind made up of particles. The energetic rays interact
with the escaped atmosphere (now we could call it the Nebula), making
it shine in many different colours which we then can observe from Earth.
The fast wind from the leftover core also interacts with the nebulae,
giving rise to the formation of many characteristic nebulae structures. As
time goes by, the nebula escapes further out in the interstellar media and
the core slowly begins to cool down and become a white dwarf (see a previous
article by Javier
on supernovae in this section). Eventually, the Nebulae is so far away from the
white dwarf that it no longer receives sufficient energy to keep shining,
and thus fades away. The nebulae can keep shining maybe up to
10-20.000 years. This seems like a long time but compared to the total
lifetime of a star, which for the Sun is believed to be around 12 thousand
million years, it is very, very short.
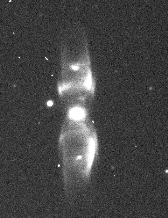 |
Butterfly Nebula. This is a picture of the Planetary
Nebulae M2-9, also called the Butterfly Nebula, taken using the 2.5m Nordic
Optical Telescope (NOT) on the Roque de Los Muchachos Observatory, and a
filter centred at the Oxygen I wavelength. Peter and
his colleagues now believe that this morphology is due to a binary system of
stars. This
picture is a courtesy of Hugo Schwarz (NOT).
|
The scientific importance of PNe is manifold. Maybe most important is
the fact that the nebula which is expelled into the interstellar media
carries with it many of the heavy elements like carbon and oxygen. These
elements were formed in the centre of the star, just before it ejected the
nebulae. As new stars and planets are formed out of the interstellar media,
their composition are enriched with heavy elements. Without this
enrichment process, the Earth would never have had enough of the heavy
elements to allow for the creation of life. Observing PNe has also proved
to be a way to determine distances to other galaxies. PNe are like candles;
When looking at a candle, one can get a pretty good estimate of the
distance to the candle. This is because everybody knows how bright a
candle really, is, and that all candles more or less have the same
brightness. Using PNe this way, distances of many galaxies has been
found. Another area in PNe research is the study of nebular structure, or
morphology. This can give information about the past evolution of these
objects, specially when at the same time it is possible to obtain data from
the central star (the core that was left over). The reason for this interest
is
that it is very difficult to explain the magnificent morphology seen in
many PNe from the standard theory described above, which for most
cases predicts a high degree of symmetry. Many theories for explaining
the observed morphology have been developed in recent years. The
most promising idea, which a group of researchers at the Roque de los
Muchachos are currently investigating, is the effects of binary systems in
the PNe formation. A binary system is two stars orbiting round each other, just
like the Earth orbiting around the Sun. We believe that the effect of such a
system would be that most of the nebulae mass will be ejected only in one
particular direction. The resulting nebulae morphology would under these
circumstances have a very characteristic 'butterfly', shape.
To prove this, we are undertaking a major survey of PNe to look
for binarity. This is done by using the 2.5m Isaac Newton Telescope
(INT) on the Roque de los Muchachos.
|