Telescope Operation
During the two-year period 2004/05 covered by this report, the ING telescopes
again performed very well, with downtime figures due to technical problems
averaging only 2.3% and 1.5%, on the William Herschel Telescope (WHT) and
the Isaac Newton Telescope (INT), respectively. These figures are well below
the target value of a maximum of 5 percent technical downtime. Observing time
lost due to poor weather over the same period averaged 34%.
Day-to-day telescope operations support is carried out by a dedicated Operations
Team, taking responsibility for the telescopes and associated infrastructure.
Efforts of day-time and night-time support activities concentrate on the WHT.
On this telescope five common-user instruments are supported, one of which
is the complex adaptive optics suite. Also several visiting instruments were
supported at the WHT. Many observing teams visit the telescopes every year.
The INT, in contrast, operates in a much simpler fashion and is a single-instrument
facility. The 1-m JKT does not host science observations anymore, but is now
regularly being used for measuring the atmospheric turbulence profile above
the observatory using the technique of SCIntillation Detection And Ranging
(SCIDAR) led by the IAC.
At night a telescope operator in always present on the WHT to assist the scientists
in taking the observations. ING’s team of astronomers acts as the primary
contact for visiting scientists. They assist visitors in taking the observations
and in carrying out observations in service for the community.
The year 2005 marked the end of an important reorganisation that was the result
of a phased reduction of operating cost of the observatory. These measures
unavoidably had an impact on the service delivered to the visiting astronomers,
but the strong focus on the operation of the WHT has meant that a continued
high level of service and flexibility could still be offered to the community
of users.
Adaptive optics observations are gradually becoming more important. The advent
of the laser guide star system in the near future will drastically enhance
the scientific potential and hence likely increase the interest in the use
of adaptive optics further. In order to ensure optimal scientific use of the
best seeing periods for adaptive optics observations the observing programme
at night must be of a flexible nature. The expectation is that during certain
periods of the year the WHT will be operated in such queue-scheduled mode.
The presence of the Isaac Newton Group of Telescopes at the Spanish Observatorio
de Roque de los Muchachos (ORM) is secured under international agreements.
These agreements will be up for renewal around the turn of the decade after
having been in force for 30 years. Leading up to this date discussions between
the various international partners are under way on how best to adapt and
continue with the formal structure in order to prepare the wider observatory
for the future. In parallel with these discussions, ideas have further matured
on potential further collaboration between telescope groups at the observatory,
building on the successful collaboration that already exists with the Italian
3.5-m Telescopio Nazionale Galileo (TNG).
Together with the international agreements also the telescope facilities advance
in age. One of the first telescopes at the ORM was the INT. In February 2004
that telescope had been in operation on La Palma for 20 years. An impressive
period of two decades of continuous operation that has resulted in many important
discoveries and well over 1100 papers published in scientific journals.
Instrumentation
An important development for the WHT was the commissioning and first science
operation of the intermediate resolution IR spectrograph and imager, LIRIS,
that was built by the Instituto de Astrofísica de Canarias. This instrument,
based on a 1024 by 1024 pixel Hawaii array detector operates in the Cassegrain
focus of the telescope and allows high quality imaging and multi-object spectroscopy
at near IR wavelengths. Following extensive testing LIRIS was taken into operation
during the summer of 2004 as a common-user instrument. The science projects
carried out with this instrument are diverse and include distant galaxies,
stellar populations, brown dwarfs and planetary nebulae, showing the potential
of this instrument for the community of users. In particular the possibility
of using multi-object masks offers a potential that is currently a rare feature
at other telescopes.
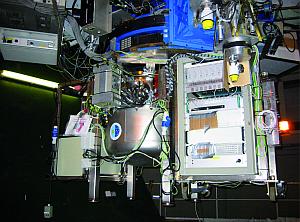 |
Figure 31. LIRIS mounted in the Cassegrain focus
of the WHT [ JPEG | TIFF
].
|
Since its commissioning LIRIS has become one of the most popular instruments
on the WHT. Its multi-slit mask mode is particularly popular as it offers
a huge multiplex advantage over classical long-slit spectroscopy and is therefore
very efficient. Many spectra of for instance galaxies in distant clusters
can be observed at the same time. Also during the year the polarisation module
was commissioned in LIRIS, offering imaging polarimetry at infrared wavelengths.
Development work at the ING has focussed on the Adaptive Optics (AO) instrumentation
suite, an extensive and rather complex set of instruments that permanently
occupies one of the Nasmyth foci at the WHT. The Adaptive Optics system itself,
NAOMI, has been in operation for four years now. It feeds three science instruments:
the infra-red imager INGRID, the coronagraph OSCA, and the optical integral
field spectrograph OASIS. The latter is the most recent addition to the AO
capability through a collaboration with the Observatoire de Lyon, France.
The OASIS adaptive-optics assisted integral field spectrograph was taken fully
into operation during the reporting period. The spectrograph works in the optical
wavelength range and offers a wide variety of spectral modes. Also optical imaging
can be conducted with this system, and best images achieved so far have been
those of a close binary system, achieving 0.1 arcsecond image width in the
I
band.
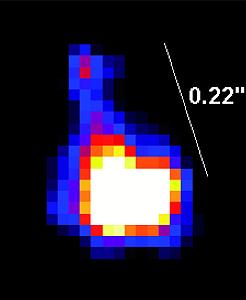 |
Figure 32. I-band image of a close binary star
with the NAOMI Adaptive Optics system using the OASIS integral field
spectrograph. [ JPEG | TIFF
].
|
The OASIS spectrograph supports a wide choice in bandwidth and resolution. OASIS
competes for photons with the wavefront sensor, and in order to have the best
possible throughput to both OASIS and the wavefront sensor a range of dichroic
beam splitters is available to pre-select the wavelength range of interest.
The dichroic reflects the appropriate waveband into OASIS while the remaining
photons are used for wavefront sensing. Until recently manually changing dichroic
filters posed a significant operational overhead, and therefore an automated
system was designed and built to swap dichroics in and out of the beam in a
fast, accurate, and efficient manner. This dichroic changer was commissioned
during the reporting period and is currently in full operation.
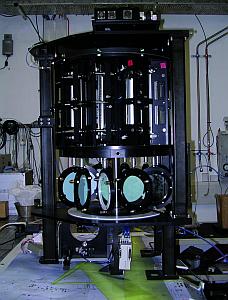 |
Figure 33. The automatic dichroic filter changer
unit [ JPEG | TIFF
].
|
Natural guide star adaptive optics is largely limited by the availability
of stars bright enough to measure the wavefront distortions on that are induced
by the Earth’s atmosphere. Measurement and correction of the wavefront
distortions has to take place in the shortest possible time in order to generate
the best results. However, short exposure times (milliseconds) also imply
that few photons are available and hence bright stars are required to generate
sufficient signal-to-noise. Also in the case of NAOMI this is a limitation
for effective and broad scientific use of the instrumentation. Being able
to detect fainter stars would help ease the situation. At very faint signals
the detector read noise becomes an important factor, and therefore a project
is under way to install a zero-read noise CCD in the existing wavefront sensor.
For faint guide stars the nearly zero read noise from this new detector is
expected to improve the detection efficiency of the system by at least a factor
of two.
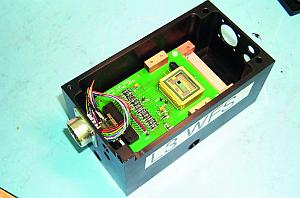 |
Figure 34. The Low-Light-Level CCD that will be
deployed as wavefront sensor in the AO system. The actual CCD chip
is the tiny black square in the yellow enclosure [ JPEG
| TIFF ].
|
The ultimate solution for finding bright enough guide stars is to create an
artificial ‘star’ that can be used as a wavefront sensor source.
Such artificial source can be created using a strong, well collimated laser
beam that is aligned with the main telescopes. Laser light that is back scattered
from high in the atmosphere can then be used as an artificial point source
suitable for wavefront sensing. In 2004 a project was initiated to create
such laser beacon based on Rayleigh back scatter from an altitude of about
20km above the observatory. This project, listening to the Dutch acronym GLAS,
for (translated) Ground-layer Laser Adaptive optics System, will work in conjunction
with the NAOMI instrument as well as with the existing science cameras.
The scientific advantages of GLAS are potentially huge as such a laser guide
star system will amplify the fraction of sky available to adaptive optics
observations at visible and infrared wavelengths from a few percent to nearly
100%. In terms of astronomical research, this translates into radical progress
as it opens up high spatial resolution observations from the ground to nearly
all types of science targets, making possible the observations of faint and
extended sources, and enabling observations of large samples, unbiased by
the fortuitous presence of nearby bright stars. In combination with the existing
instrumentation the WHT will offer a highly competitive facility to the astronomical
community, exploiting a window of opportunity before similar capability will
exist on 8-m class telescopes.
The system design of GLAS was fully completed in 2005. At the heart of the
system will be a 515nm green laser of about 30W. This laser will be mounted
at the top of the telescope. Its light beam will travel to the centre of the
telescope, behind the secondary mirror, where it will be injected into a beam
launch telescope. There the laser beam will be expanded to about 35cm, and
subsequently projected into the atmosphere where it will be focussed at a
distance of 20km. The diagram below gives a good impression of what this part
of the system will look like once installed at the WHT. Expectation is that
the laser system will be commissioned in 2006.
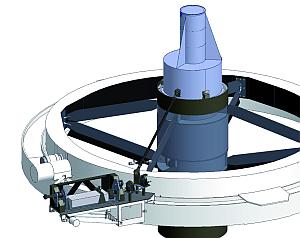 |
Figure 35. Top ring of the WHT, with shown in the
front the laser unit inside a cradle. Laser light will travel through
an evacuated pipe to the centre of the telescope, where it will be
projected into the atmosphere by the beam launch telescope that is
located behind the secondary mirror [ JPEG
| TIFF ].
|
Existing instruments remain competitive through the continuous upgrade and
improvement of its components. Therefore, apart from the major new instrument
developments mentioned above, there have also been a wide range of enhancements
to instruments, all with the aim to improve the scientific capability and
operational efficiency. An important milestone was achieved with the successful
commissioning of the WYFFOS long-camera. This new camera to the fibre-fed
WYFFOS spectrograph has the advantages over the original camera that it accommodates
a larger number of fibres, has an external focus permitting change of detector,
and provides a somewhat higher spectral resolution than the previous camera.
The camera was built by the ASTRON institute in the Netherlands.
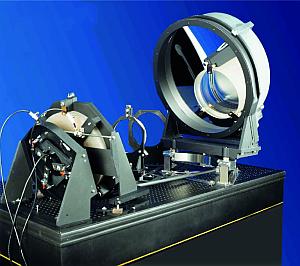 |
Figure 36. WYFFOS long camera [ JPEG
| TIFF ].
|
Apart from the hardware changes for the WYFFOS instruments, there have also
been very significant upgrades of the software. In particular the software
responsible for setting up the multiple fibre module, AUTOFIB, that feeds
WYFFOS has seen major modernization, resulting, amongst other things, in a
drastic reduction of the fibre setup time.
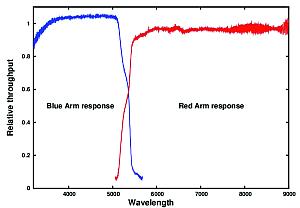 |
Figure 37. Response curve of the new dichroic of
the ISIS spectrograph [ JPEG | TIFF
].
|
Also the venerable ISIS spectrograph has seen important advances with the
acquisition of a new dichroic filter that splits light between the red and
blue optimized spectrograph arms. The new dichroic possesses a sharper cut
between the wavelength ranges at a wavelength that is appropriate for most
science programmes, and also features a smoother response. A small but very
significant upgrade that has helped the efficiency of the instrument as a
whole.
The WHT remains very popular with university groups who build dedicated instruments
to find answers to specific astronomical questions. The infrastructure and
support offered by ING greatly assists the exploitation of visiting instruments.
For that reason over the years many instruments have been deployed in this
way and during the reporting year no less than six different visiting instruments
came to the telescope. New instruments that had not been to the WHT before
were the CIRPASS multi-object IR spectrograph and PLANETPOL, capable of extremely
high accuracy polarimetric observations. A brief description of these two
new visiting instruments follows.
CIRPASS is a near-IR fibre-fed cooled spectrograph, designed and built by
a team from the University of Cambridge. The acronym stands for the Cambridge
Infra-Red Panoramic Survey Spectrograph. CIRPASS can take either an integral
field fibre bundle or a multi-object fibre bundle. The latter was used at
the WHT. A plug-plate system of pre-drilled holes allowed the acquisition
and observation of many galaxies simultaneously, thus providing a huge multiplex
advantage. The observations focussed on the star formation rates in distance
galaxies such as those found in the Hubble Deep Field.
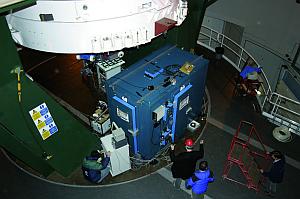 |
Figure 38. The CIRPASS IR spectrograph (blue cabinet)
being positioned on the moving platform at the base of the WHT. Light
from the Cassegrain focus is fed through a large bundle of fibres
to the spectrograph [ JPEG | TIFF
].
|
The second visiting instrument new to the WHT was PLANETPOL, from the University
of Hertfordshire (UK). The key objective of this instrument is to detect and
characterise scattered light from planets around distant stars. Starlight
scattered on the surface and in the atmospheres of extra-solar planets causes
a weak but characteristic polarization signal. PLANETPOL is designed to detect
such very weak signals and has already proven it can achieve that demanding
goal.
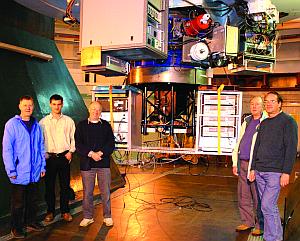 |
Figure 39. The PLANETPOL instrument mounted in
the Cassegrain focus of the WHT, together with the team of scientists
[ JPEG | TIFF ].
|
The WHT also keeps attracting experimental activities. Arguably the most exciting
set of experiments relate to the future development of extremely large telescopes.
The future telescopes will have segmented primary mirrors and a very significant
and costly problem is the control of the segments in order to keep them aligned
at all times to a fraction of a wavelength. Of course this problem has been
solved by existing segmented telescopes such as the Keck twins on Hawaii and
the GTC on La Palma, but for future ELTs the problem is much more severe and
costly. Therefore alternative methods are being considered to determine the
phase difference between the segments by optical means, in a fast way, and
by using starlight. The results of such measurement can then be used to mechanically
control the relative position of the mirror segments.
Theoretical work by the group in Arcetri, Italy showed the feasibility using
a novel technique of the pyramid wavefront sensor, but this required testing
under real conditions as proof-of-concept. This idea inspired collaboration
with groups from Durham, UK to use the deformable mirror of the NAOMI adaptive
optics system which happens to have individual segments that can be controlled,
thus serving as a tiny prototype of a future giant telescope. The experiments
were very successful and have proven that the concept is indeed viable.
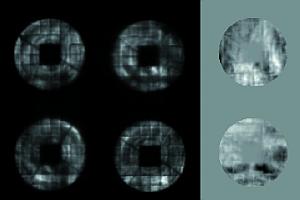 |
Figure 40. Picture of WHT telescope pupil as see
through the pyramid wavefront sensor. The resulting mirror flatness
over the controlled subset of mirror elements was better than 10nm
[ JPEG | TIFF
].
|
Another very successful experimental activity that was hosted by the ING falls
in the field of astronomical site characterization. In past years researchers
from the University of Durham had developed a novel method to measure atmospheric
turbulence. This method of Slope Detection and Ranging, or SLODAR, had been
extensively tested on the WHT and was so successful in measuring turbulence
profiles in the lower atmosphere that a portable unit was designed. This unit
would be deployed at different observatories as a site testing tool in preparation
for the future construction of extremely large telescopes. First tests were
carried out on La Palma, hosted by the ING.
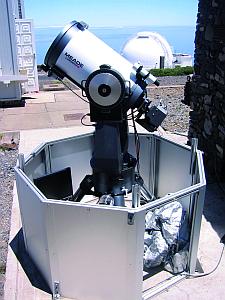 |
Figure 41. First deployment of the SLODAR equipment
[ JPEG | TIFF ].
|
Infrastructure
The LIRIS IR spectrograph was commissioned during the reporting period. LIRIS,
being a complex cryogenic instrument, requires particular care also when it
is not mounted on the telescope. For that reason a dedicated 'parking' area
was constructed in the basement of the WHT. In this enclosure the instrument
can be kept cold and connected to the network and ancillary equipment for monitoring
and control. Furthermore, a mobile, deployable clean room has been acquired
that can be installed in the aluminizing area. The prime purpose of this acquisition
was for LIRIS in case the instrument needs to be opened up, but it will be equally
useful for similar operations on other instruments.
An important milestone was reached with the full modernization of the detector
controller infrastructure. Not only the science detectors, but also all TV and
autoguider systems now operate on the basis on SDSU controllers while data acquisition
runs through ING's ULTRADAS software system. Having a uniform infrastructure
for these systems offers significant advantages for maintenance, while at the
same time performance of these modern systems is much better.
Steady progress was made on installation of a higher bandwidth data link between
the Mayantigo office building at sea level and the observatory site, culminating
in an order-of-magnitude enhancement. Also the connection of the observatory
to the Internet improved drastically. This improvement has meant, amongst other
things, that archiving of scientific data in Cambridge is now automated and
does not require posting of tapes or disks anymore.
Over the years ING has been making steady progress in tracking and implementing
the latest CCD detector technologies. Activities include the use of low-light-level
CCDs for wavefront sensing as well as for high speed spectroscopy, optimized
coatings to reduce fringing at long wavelengths, and testing of surface treatment
techniques also to reduce fringing.
A low-key activity has been the step-wise improvement to the WHT control room,
in order to make it a more friendly work environment, including new furniture,
additional possibilities for computer connections, and improvements in lighting,
computer screens and insulation from the somewhat noisy electronics cabinets.
During the reporting period both the INT and WHT primary mirrors were re-aluminized.
Also mirrors from partner telescopes at the observatory make use of INGs experience
and infrastructure. The past two years both the mirror of the Nordic Optical
Telescope and of the Liverpool Telescope were aluminized by INGs team.
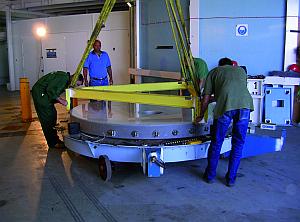 |
Figure 42. Lifting of primary mirror of the Nordic
Optical Telescope in preparation for aluminizing [ JPEG
| TIFF ].
|
Early in the reporting period the SUPERWASP experiment, led by the Queens University
Belfast and hosted by the ING was officially inaugurated. The installation consists
of a number of wide-field cameras on a robotic mount, located in an automatic
roll-off roof enclosure. The main scientific aim of this experiment is the detection
of planets around stars through their occulting effect when the planet transits
the stellar surface. The inauguration event was carried out, very appropriately,
by remote control; the mayor of the municipality of Garafía where the
observatory is located, Mr. José Peñate, commanded the instrument
to move and cut a red ribbon.
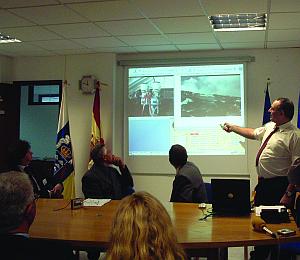 | 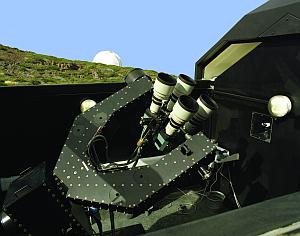 |
Figure 43. Left: SuperWASP inauguration by remote
control [ JPEG | TIFF
]. Right: Enclosure open of SuperWASP and WHT dome in the background
[ JPEG | TIFF ].
|